Detailed calculations reveal a surprise about how black holes bend light around themselves, opening a new possibility for future observations.
By now, nearly all of us have seen the Event Horizon Telescope’s iconic image of a black hole. The supermassive black hole sits at the center of the galaxy M87 in the constellation Virgo, happily chomping down on a light diet of hot gas. Photons radiated from this gas and from elsewhere loop around the black hole just outside the event horizon, the infamous point of no return. These photons outline the event horizon’s round silhouette to create the glowing halo and black hole “shadow” shown in the image.
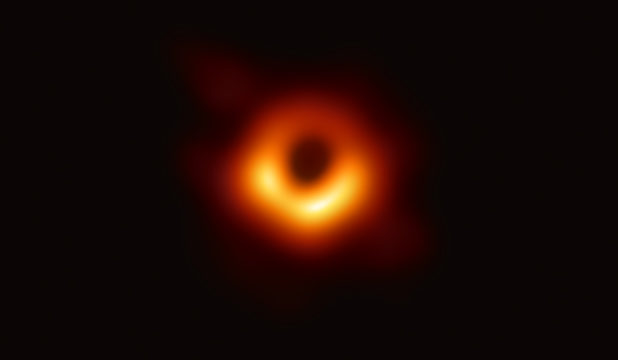
EHT Collaboration
The inner edge of the silhouette’s bright halo is called the photon ring. It marks the closest circuit light can make around the black hole without plunging into it. Einstein’s general theory of relativity predicts the ring’s size and shape, and many have hailed the M87 image as a verification of Einstein’s gravity.
But it seems we did not understand this ring as well as we thought we did. Calculations by a team at Harvard’s Black Hole Initiative and their colleagues show that for spinning black holes, the photon ring is actually not a single ring. Instead, it’s an infinite series of concentric “subrings,” all piled up together, each one thinner than the one just outside it. Careful observations may enable us to use the subrings as a unique window, shining light (pun intended) on the way the black hole warps the fabric of spacetime around it.
How Radio Dishes Could Detect Subrings
The photons that trace out the subrings are temporary hostages of the black hole, their trajectories diverted by its gravity. A photon winds up in a particular subring based on how many half orbits it completed around the black hole before escaping to reach us. The more loops completed, the thinner the subring, and the closer it lies to the event horizon in the image.
How these subrings change in thickness, shape, and brightness as you go from one subring to the next depends on the way the black hole warps spacetime around itself. This geometry in turn depends on the black hole’s mass and spin. So studying the subrings could not only give us a new way to “see” the gravitational landscape but also tell us the two properties that define a black hole.
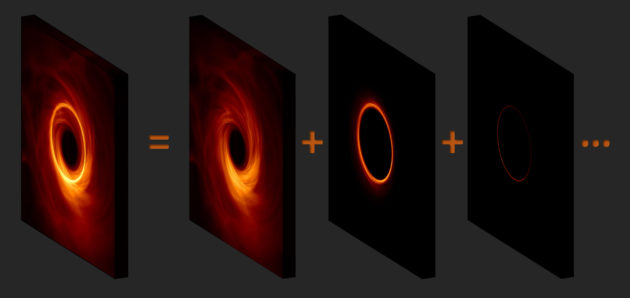
Michael D. Johnson (CfA) and George Wong (UIUC)
This is where the Event Horizon Telescope’s global network of radio telescopes comes in. The EHT observes using a technique called very long baseline interferometry, or VLBI. It combines observations taken by multiple pairs of telescopes to reconstruct an image. Each pair of radio dishes detects stuff at a different scale depending on the dishes’ separation, called a baseline. Longer baselines pick up smaller structure.
Right now, the EHT only detects a puffy photon ring around M87’s black hole. But if researchers extend the array into space, they would have long enough baselines to start accessing the ring’s thickness, explains coauthor Michael Johnson (Center for Astrophysics, Harvard & Smithsonian). And because each subring is a different thickness, each baseline will only pick up photons from a given subring and the ones narrower than it, which stack together in the data like a tiered wedding cake, the team writes March 18th in Science Advances.
With two space-based telescopes — say, a geosynchronous satellite and a station on the Moon — the EHT could detect where different subrings disappear. It could also measure the photon ring’s diameter, which gives the black hole’s mass. If the photon ring is not perfectly circular but squashed, that could tell astronomers the black hole’s spin.
The subrings are a unique prediction of general relativity that languished undiscovered for decades, say Johnson and Alexandru Lupsasca (Harvard). After they came up with the rough idea, Lupsasca spent a couple of months with a collaborator, working through the grueling calculations. The results confirmed their subrings hypothesis.
“It’s a great paper because it treats the problem from all angles,” says theorist Samuel Gralla (University of Arizona) — new theory, new simulations showing the subrings, and new ideas for observing them in nature, he explains. Gralla and his colleagues had been thinking about subrings in vaguer terms around the same time as Johnson and Lupsasca’s team, and since then he’s teamed up with Lupsasca to dive into the details.
Center for Astrophysics, Harvard & Smithsonian
Around the Photon Ring
There’s a second way subrings may pull back the curtain on black holes. To understand it, forget for a moment the cascade of concentric rings and just visualize one.
A photon ring is a combination of light that traveled in unstable orbits of different sizes around the black hole. But the orbiting photons don’t travel in simple arcs or circles, Johnson says. A spinning black hole drags spacetime around with it, and the photons must navigate this wacky landscape, following twisted paths that pull them first one way, then another as they loop around to the black hole’s other side. The trajectories wind up looking like contorted rubber bands.
The photons can escape anywhere along their path, shooting away in random directions. But if you’re looking at the black hole, you won’t see all those photons — you’ll only see the ones that reach you. These will come at you from one of two exit points. The location of these points depends on the radius of the photon’s orbit around the black hole. All photons from a given orbit would reach you from the same two escape hatches. Photons from a different orbit will come in at a different angle.
These different exit points are like tick marks on the edge of a clockface. By themselves they’re just points in space, but add them all up and they draw a ring around the black hole — the photon ring. Essentially, the photon ring is like a hologram, mapping the 3D structure of spacetime around the black hole into a 2D shape, Johnson says. The implication is that astronomers can look at a point on the photon ring and know exactly how those photons were traveling around the black hole before they escaped.
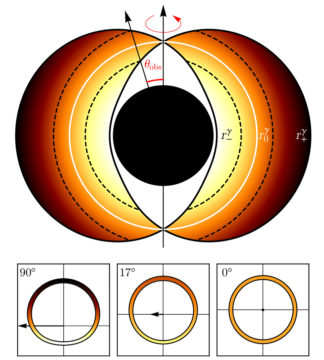
M. D. Johnson et al. / Sci Adv 2020 CC BY-NC 4.0
Now add the other subrings back in. Remember that each subring is made of light that traveled a different number of times around the black hole before flying away. So if astronomers look at the same section — say, the six o’clock point — in different subrings, they’ll be looking at different snapshots of photons in the same orbit. They can then see how the light in that orbit changed with time. Any changes would tell us about what the black hole is doing to the surrounding spacetime as it whirls around.
Reference: M. D. Johnson et al. “Universal Interferometric Signatures of a Black Hole’s Photon Ring.” Science Advances. March 18, 2020.
Comments
You must be logged in to post a comment.